Full Paper View Go Back
Conceptual Framework and Advances in CRISPR-Cas Based and BRED Phage Engineering
Riya Manas Sharma1
Section:Review Paper, Product Type: Journal-Paper
Vol.8 ,
Issue.4 , pp.1-5, Aug-2021
Online published on Aug 31, 2021
Copyright © Riya Manas Sharma . This is an open access article distributed under the Creative Commons Attribution License, which permits unrestricted use, distribution, and reproduction in any medium, provided the original work is properly cited.
View this paper at Google Scholar | DPI Digital Library
How to Cite this Paper
- IEEE Citation
- MLA Citation
- APA Citation
- BibTex Citation
- RIS Citation
IEEE Style Citation: Riya Manas Sharma, “Conceptual Framework and Advances in CRISPR-Cas Based and BRED Phage Engineering,” International Journal of Scientific Research in Biological Sciences, Vol.8, Issue.4, pp.1-5, 2021.
MLA Style Citation: Riya Manas Sharma "Conceptual Framework and Advances in CRISPR-Cas Based and BRED Phage Engineering." International Journal of Scientific Research in Biological Sciences 8.4 (2021): 1-5.
APA Style Citation: Riya Manas Sharma, (2021). Conceptual Framework and Advances in CRISPR-Cas Based and BRED Phage Engineering. International Journal of Scientific Research in Biological Sciences, 8(4), 1-5.
BibTex Style Citation:
@article{Sharma_2021,
author = {Riya Manas Sharma},
title = {Conceptual Framework and Advances in CRISPR-Cas Based and BRED Phage Engineering},
journal = {International Journal of Scientific Research in Biological Sciences},
issue_date = {8 2021},
volume = {8},
Issue = {4},
month = {8},
year = {2021},
issn = {2347-2693},
pages = {1-5},
url = {https://www.isroset.org/journal/IJSRBS/full_paper_view.php?paper_id=2466},
publisher = {IJCSE, Indore, INDIA},
}
RIS Style Citation:
TY - JOUR
UR - https://www.isroset.org/journal/IJSRBS/full_paper_view.php?paper_id=2466
TI - Conceptual Framework and Advances in CRISPR-Cas Based and BRED Phage Engineering
T2 - International Journal of Scientific Research in Biological Sciences
AU - Riya Manas Sharma
PY - 2021
DA - 2021/08/31
PB - IJCSE, Indore, INDIA
SP - 1-5
IS - 4
VL - 8
SN - 2347-2693
ER -
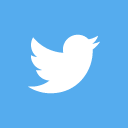
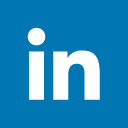
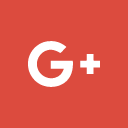
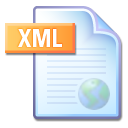
Abstract :
The increased proliferation of multi-antibiotic resistant bacterial pathogens necessitates the development of alternate and supportive therapies to antibiotics. Being natural predators of bacteria, bacteriophages have garnered recent attention for their untapped potential to mitigate the public health problem. Progress in synthetic biology has led to the genetic engineering of phages to ameliorate their efficacy and precision via methods such as genome editing, modification of tail fibers, and treatment with chemical mutagens. This review will elucidate two promising genetic engineering strategies, CRISPR-Cas and Bacteriophage Recombineering of Electroporated DNA (BRED), as well as the advances and potential future developments in the field.
Key-Words / Index Term :
Bacteriophages, Genetic Engineering, CRISPR-Cas systems, BRED Phage Engineering
References :
[1] G. F. Hatfull, “Bacteriophage genomics,” Current Opinion in Microbiology, vol. 11, no. 5, Oct. 2008, doi: 10.1016/j.mib.2008.09.004.
[2] L. J. Piddock, “The crisis of no new antibiotics—what is the way forward?,” The Lancet Infectious Diseases, vol. 12, no. 3, Mar. 2012, doi: 10.1016/S1473-3099(11)70316-4.
[3] D. M. Lin, B. Koskella, and H. C. Lin, “Phage therapy: An alternative to antibiotics in the age of multi-drug resistance,” World Journal of Gastrointestinal Pharmacology and Therapeutics, vol. 8, no. 3, 2017, doi: 10.4292/wjgpt.v8.i3.162.
[4] R. T. Schooley et al., “Development and Use of Personalized Bacteriophage-Based Therapeutic Cocktails To Treat a Patient with a Disseminated Resistant Acinetobacter baumannii Infection,” Antimicrobial Agents and Chemotherapy, vol. 61, no. 10, Oct. 2017, doi: 10.1128/AAC.00954-17.
[5] A. P. Sagona, A. M. Grigonyte, P. R. MacDonald, and A. Jaramillo, “Genetically modified bacteriophages,” Integrative Biology, vol. 8, no. 4, 2016, doi: 10.1039/C5IB00267B.
[6] R. Marzari, D. Sblattero, M. Righi, and A. Bradbury, “Extending filamentous phage host range by the grafting of a heterologous receptor binding domain,” Gene, vol. 185, no. 1, Jan. 1997, doi: 10.1016/S0378-1119(96)00623-3.
[7] K. Yehl et al., “Engineering Phage Host-Range and Suppressing Bacterial Resistance through Phage Tail Fiber Mutagenesis,” Cell, vol. 179, no. 2, Oct. 2019, doi: 10.1016/j.cell.2019.09.015.
[8] P. Lucchesi, M. Carraway, and M. G. Marinus, “Analysis of forward mutations induced by N-methyl-N’-nitro-N-nitrosoguanidine in the bacteriophage P22 mnt repressor gene,” Journal of Bacteriology, vol. 166, no. 1, Apr. 1986, doi: 10.1128/jb.166.1.34-37.1986.
[9] A. M. Grigonyte et al., “Comparison of CRISPR and Marker-Based Methods for the Engineering of Phage T7,” Viruses, vol. 12, no. 2, Feb. 2020, doi: 10.3390/v12020193.
[10] M. M. Jore, S. J. J. Brouns, and J. van der Oost, “RNA in Defense: CRISPRs Protect Prokaryotes against Mobile Genetic Elements,” Cold Spring Harbor Perspectives in Biology, vol. 4, no. 6, Jun. 2012, doi: 10.1101/cshperspect.a003657.
[11] J. Bondy-Denomy, A. Pawluk, K. L. Maxwell, and A. R. Davidson, “Bacteriophage genes that inactivate the CRISPR/Cas bacterial immune system,” Nature, vol. 493, no. 7432, Jan. 2013, doi: 10.1038/nature11723.
[12] K. S. Makarova et al., “Evolutionary classification of CRISPR–Cas systems: a burst of class 2 and derived variants,” Nature Reviews Microbiology, vol. 18, no. 2, Feb. 2020, doi: 10.1038/s41579-019-0299-x.
[13] A. Hatoum-Aslan, “Phage Genetic Engineering Using CRISPR–Cas Systems,” Viruses, vol. 10, no. 6, Jun. 2018, doi: 10.3390/v10060335.
[14] R. Kiro, D. Shitrit, and U. Qimron, “Efficient engineering of a bacteriophage genome using the type I-E CRISPR-Cas system,” RNA Biology, vol. 11, no. 1, Jan. 2014, doi: 10.4161/rna.27766.
[15] A. A. Gomaa, H. E. Klumpe, M. L. Luo, K. Selle, R. Barrangou, and C. L. Beisel, “Programmable Removal of Bacterial Strains by Use of Genome-Targeting CRISPR-Cas Systems,” mBio, vol. 5, no. 1, Feb. 2014, doi: 10.1128/mBio.00928-13.
[16] B. Martel and S. Moineau, “CRISPR-Cas: an efficient tool for genome engineering of virulent bacteriophages,” Nucleic Acids Research, vol. 42, no. 14, Aug. 2014, doi: 10.1093/nar/gku628.
[17] M. Jinek, K. Chylinski, I. Fonfara, M. Hauer, J. A. Doudna, and E. Charpentier, “A Programmable Dual-RNA-Guided DNA Endonuclease in Adaptive Bacterial Immunity,” Science, vol. 337, no. 6096, Aug. 2012, doi: 10.1126/science.1225829.
[18] K. S. Makarova, N. v Grishin, S. A. Shabalina, Y. I. Wolf, and E. v Koonin, “A putative RNA-interference-based immune system in prokaryotes: computational analysis of the predicted enzymatic machinery, functional analogies with eukaryotic RNAi, and hypothetical mechanisms of action,” Biology Direct, vol. 1, no. 1, 2006, doi: 10.1186/1745-6150-1-7.
[19] S. J. J. Brouns et al., “Small CRISPR RNAs Guide Antiviral Defense in Prokaryotes,” Science, vol. 321, no. 5891, Aug. 2008, doi: 10.1126/science.1159689.
[20] L. J. Marinelli et al., “BRED: A Simple and Powerful Tool for Constructing Mutant and Recombinant Bacteriophage Genomes,” PLoS ONE, vol. 3, no. 12, Dec. 2008, doi: 10.1371/journal.pone.0003957.
[21] D. L. Court, J. A. Sawitzke, and L. C. Thomason, “Genetic Engineering Using Homologous Recombination,” Annual Review of Genetics, vol. 36, no. 1, Dec. 2002, doi: 10.1146/annurev.genet.36.061102.093104.
[22] K. C. Murphy, “Use of Bacteriophage ? Recombination Functions To Promote Gene Replacement in Escherichia coli,” Journal of Bacteriology, vol. 180, no. 8, Apr. 1998, doi: 10.1128/JB.180.8.2063-2071.1998.
[23] D. Yu, H. M. Ellis, E.-C. Lee, N. A. Jenkins, N. G. Copeland, and D. L. Court, “An efficient recombination system for chromosome engineering in Escherichia coli,” Proceedings of the National Academy of Sciences, vol. 97, no. 11, May 2000, doi: 10.1073/pnas.100127597.
[24] H. M. Ellis, D. Yu, T. DiTizio, and D. L. Court, “High efficiency mutagenesis, repair, and engineering of chromosomal DNA using single-stranded oligonucleotides,” Proceedings of the National Academy of Sciences, vol. 98, no. 12, Jun. 2001, doi: 10.1073/pnas.121164898.
[25] S. Datta, N. Costantino, and D. L. Court, “A set of recombineering plasmids for gram-negative bacteria,” Gene, vol. 379, Sep. 2006, doi: 10.1016/j.gene.2006.04.018.
[26] Y. Zhang, F. Buchholz, J. P. P. Muyrers, and A. F. Stewart, “A new logic for DNA engineering using recombination in Escherichia coli,” Nature Genetics, vol. 20, no. 2, Oct. 1998, doi: 10.1038/2417.
[27] J. P. P. Muyrers, Y. Zhang, and A. F. Stewart, “Techniques: Recombinogenic engineering–new options for cloning and manipulating DNA,” Trends in Biochemical Sciences, vol. 26, no. 5, May 2001, doi: 10.1016/S0968-0004(00)01757-6.
[28] D. Manna, S. Deng, A. M. Breier, and N. P. Higgins, “Bacteriophage Mu Targets the Trinucleotide Sequence CGG,” Journal of Bacteriology, vol. 187, no. 10, May 2005, doi: 10.1128/JB.187.10.3586-3588.2005.
[29] A. B. Oppenheim, A. J. Rattray, M. Bubunenko, L. C. Thomason, and D. L. Court, “In vivo recombineering of bacteriophage ? by PCR fragments and single-strand oligonucleotides,” Virology, vol. 319, no. 2, Feb. 2004, doi: 10.1016/j.virol.2003.11.007.
[30] L. J. Marinelli, G. F. Hatfull, and M. Piuri, “Recombineering,” Bacteriophage, vol. 2, no. 1, Jan. 2012, doi: 10.4161/bact.18778.
[31] S. K. Sharan, L. C. Thomason, S. G. Kuznetsov, and D. L. Court, “Recombineering: a homologous recombination-based method of genetic engineering,” Nature Protocols, vol. 4, no. 2, Feb. 2009, doi: 10.1038/nprot.2008.227.
[32] D. P. Pires, S. Cleto, S. Sillankorva, J. Azeredo, and T. K. Lu, “Genetically Engineered Phages: a Review of Advances over the Last Decade,” Microbiology and Molecular Biology Reviews, vol. 80, no. 3, Sep. 2016, doi: 10.1128/MMBR.00069-15.
[33] W. C. Summers, “Bacteriophage Therapy,” Annual Review of Microbiology, vol. 55, no. 1, Oct. 2001, doi: 10.1146/annurev.micro.55.1.437.
[34] T. M. Viertel, K. Ritter, and H.-P. Horz, “Viruses versus bacteria--novel approaches to phage therapy as a tool against multidrug-resistant pathogens,” Journal of Antimicrobial Chemotherapy, vol. 69, no. 9, Sep. 2014, doi: 10.1093/jac/dku173.
[35] F. L. Gordillo Altamirano and J. J. Barr, “Phage Therapy in the Postantibiotic Era,” Clinical Microbiology Reviews, vol. 32, no. 2, Mar. 2019, doi: 10.1128/CMR.00066-18.
[36] A. M. Box, M. J. McGuffie, B. J. O’Hara, and K. D. Seed, “Functional Analysis of Bacteriophage Immunity through a Type I-E CRISPR-Cas System in Vibrio cholerae and Its Application in Bacteriophage Genome Engineering,” Journal of Bacteriology, vol. 198, no. 3, Feb. 2016, doi: 10.1128/JB.00747-15.
[37] J. Shen, J. Zhou, G.-Q. Chen, and Z.-L. Xiu, “Efficient Genome Engineering of a Virulent Klebsiella Bacteriophage Using CRISPR-Cas9,” Journal of Virology, vol. 92, no. 17, Sep. 2018, doi: 10.1128/JVI.00534-18.
[38] P. Tao, X. Wu, W.-C. Tang, J. Zhu, and V. Rao, “Engineering of Bacteriophage T4 Genome Using CRISPR-Cas9,” ACS Synthetic Biology, vol. 6, no. 10, Oct. 2017, doi: 10.1021/acssynbio.7b00179.
[39] S. J. Yaung, K. M. Esvelt, and G. M. Church, “CRISPR/Cas9-Mediated Phage Resistance Is Not Impeded by the DNA Modifications of Phage T4,” PLoS ONE, vol. 9, no. 6, Jun. 2014, doi: 10.1371/journal.pone.0098811.
[40] F. Hoshiga, K. Yoshizaki, N. Takao, K. Miyanaga, and Y. Tanji, “Modification of T2 phage infectivity toward Escherichia coli O157:H7 via using CRISPR/Cas9,” FEMS Microbiology Letters, vol. 366, no. 4, Feb. 2019, doi: 10.1093/femsle/fnz041.
[41] S. M. N. Bari, F. C. Walker, K. Cater, B. Aslan, and A. Hatoum-Aslan, “Strategies for Editing Virulent Staphylococcal Phages Using CRISPR-Cas10,” ACS Synthetic Biology, vol. 6, no. 12, Dec. 2017, doi: 10.1021/acssynbio.7b00240.
[42] T. Fehér, I. Karcagi, F. R. Blattner, and G. Pósfai, “Bacteriophage recombineering in the lytic state using the lambda red recombinases,” Microbial Biotechnology, vol. 5, no. 4, Jul. 2012, doi: 10.1111/j.1751-7915.2011.00292.x.
[43] J. L. da Silva et al., “Application of BRED technology to construct recombinant D29 reporter phage expressing EGFP,” FEMS Microbiology Letters, vol. 344, no. 2, Jul. 2013, doi: 10.1111/1574-6968.12171.
[44] S. Datta, N. Costantino, X. Zhou, and D. L. Court, “Identification and analysis of recombineering functions from Gram-negative and Gram-positive bacteria and their phages,” Proceedings of the National Academy of Sciences, vol. 105, no. 5, Feb. 2008, doi: 10.1073/pnas.0709089105.
[45] R. M. Dedrick, C. A. Guerrero Bustamante, R. A. Garlena, R. S. Pinches, K. Cornely, and G. F. Hatfull, “Mycobacteriophage ZoeJ: A broad host-range close relative of mycobacteriophage TM4,” Tuberculosis, vol. 115, Mar. 2019, doi: 10.1016/j.tube.2019.01.002.
[46] R. M. Dedrick et al., “Engineered bacteriophages for treatment of a patient with a disseminated drug-resistant Mycobacterium abscessus,” Nature Medicine, vol. 25, no. 5, May 2019, doi: 10.1038/s41591-019-0437-z.
[47] K. S. Wetzel et al., “CRISPY-BRED and CRISPY-BRIP: efficient bacteriophage engineering,” Scientific Reports, vol. 11, no. 1, Dec. 2021, doi: 10.1038/s41598-021-86112-6.
[48] “Antimicrobial Resistance: Tackling a crisis for the health and wealth of nations,” Dec. 2014. Accessed: Jul. 08, 2021. [Online]. Available: http://www.jpiamr.eu/wp-content/uploads/2014/12/AMR-Review-Paper-Tackling-a-crisis-for-the-health-and-wealth-of-nations_1-2.pdf
[49] X. Huang, X. Wang, J. Zhang, N. Xia, and Q. Zhao, “Escherichia coli-derived virus-like particles in vaccine development,” npj Vaccines, vol. 2, no. 1, Dec. 2017, doi: 10.1038/s41541-017-0006-8.
[50] P. Tao, J. Zhu, M. Mahalingam, H. Batra, and V. B. Rao, “Bacteriophage T4 nanoparticles for vaccine delivery against infectious diseases,” Advanced Drug Delivery Reviews, vol. 145, May 2019, doi: 10.1016/j.addr.2018.06.025.
[51] I. Kim, J.-S. Moon, and J.-W. Oh, “Recent advances in M13 bacteriophage-based optical sensing applications,” Nano Convergence, vol. 3, no. 1, Dec. 2016, doi: 10.1186/s40580-016-0087-5.
[52] D. M. C. Leite, X. Brochet, G. Resch, Y.-A. Que, A. Neves, and C. Peña-Reyes, “Computational prediction of inter-species relationships through omics data analysis and machine learning,” BMC Bioinformatics, vol. 19, no. S14, Nov. 2018, doi: 10.1186/s12859-018-2388-7.
[53] C. Howard-Varona, K. R. Hargreaves, S. T. Abedon, and M. B. Sullivan, “Lysogeny in nature: mechanisms, impact and ecology of temperate phages,” The ISME Journal, vol. 11, no. 7, Jul. 2017, doi: 10.1038/ismej.2017.16.
You do not have rights to view the full text article.
Please contact administration for subscription to Journal or individual article.
Mail us at support@isroset.org or view contact page for more details.